Polymerase Chain Reaction – Variations of DNA polymerase
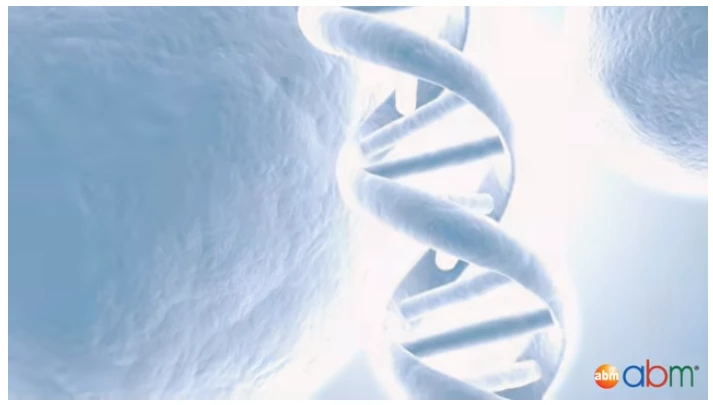
Polymerase Chain Reaction – Variations of DNA polymerase
Applied Biological Materials (abm) Inc
Contents
- Polymerase Chain Reaction – An Introduction
- Polymerase Chain Reaction – Variations of DNA polymerase
- Structure and Mechanism of Action
- Common DNA Polymerases
- Pwo DNA polymerase
- Tth polymerase
- Polymerase Chain Reaction – Real-Time PCR/ Quantitative PCR (qPCR)
- Polymerase Chain Reaction – Reverse Transcription
- Polymerase Chain Reaction – Variation to System
Structure and Mechanism of Action
All DNA polymerases are similar in shape in that they resemble a “right hand” with common structural features of a “thumb,” “palm,” and “fingers” domains. The “palm” area is the most similar among the polymerase families and is associated with catalysis of the phosphoryl transfer reaction (1). The “fingers” domain is involved in the interactions between the nucleoside triphosphate being inserted and the existing template base, and the “thumb” is suggested to assist in aligning the double-stranded DNA (1).
Primers are oligonucleotides that can bind to a specific sequence of the DNA template to guide DNA polymerase replication. When the DNA template strands dissociate, a primer with a free 3’ hydroxyl group anneals to its specific template sequence. Researchers can selectively replicate any regions of interest on the template DNA by flanking the region with specifically designed primers. Primer annealing initiates the DNA polymerase to add free nucleotides onto the hydroxyl group via a phosphoryl transfer reaction to elongate the new strand in a 5’-3’ direction. When a nucleoside triphosphate (NTP) binds to DNA polymerase, the DNA polymerase undergoes a conformational change and generates a specific shape/pocket into which only the base on the template strand and a properly shaped complementary nucleotide can fit (cytosine to guanine, thymine to adenine). In this way, DNA polymerase is able to select the correct nucleotides for incorporation. (1) (25)
Figure 1 –Mechanism of DNA extension by DNA polymerase.
Fidelity
A DNA polymerase’s fidelity refers to its accuracy during replication of the amplicon; high fidelity is achieved by having a low mis-incorporation rate as well as a proofreading mechanism. Many polymerases have a 3’-5’ exonuclease domain independent of the polymerization process, allowing them to remove mis-incorporated nucleotides from the 3' end while the DNA is being formed in the 5'-3' direction (1). Nonproofreading DNA polymerases are sufficient for routine PCR for their higher yield in general, but polymerases with proofreading properties are estimated to have 10-1000 times higher fidelity than those without (2). This has major implications for the downstream applications of PCR products for which high accuracy is crucial, such as in mutagenesis and subcloning. Furthermore, polymerases with 3’-5’ exonuclease activity result in DNA products with blunt ends as excess 3’ nucleotides are excised at the end of extension process (3). This allows for direct ligation into blunt-ended cloning vectors.
Abm's Bestaq™, Kodaq, Precision™,TaqFast and Taq Plus DNA polymerases possess 3’-5’ exonuclease activity.
Figure 2 –Mismatched bases are more likely to leave the polymerase site and migrate to the exonuclease site, where the last nucleotide added is removed by hydrolysis.
DNA polymerases can also display 5'-3' exonuclease activity, allowing for the excision of nucleotides from the 5' end of the DNA strand in a nick translation reaction (4). In real-time PCR application, this attribute is important as it cleaves labeled oligonucleotide probes from the 5' end of the DNA to generate a detectable signal (5).
Abm's Taq DNA polymerase exhibits the 5’-3’ exonuclease activity.
Conversely, some DNA polymerases lack any exonuclease activity, which allows solely for the extension of a DNA strand at the 3' end causing displacement of downstream DNA, a property that is exploited in isothermal strand displacement amplification applications (7). (Figure 3)
Figure 3
PCR originally utilized the Klenow fragment, a proteolytic product of DNA polymerase I isolated from E. coli (6). While it has high fidelity, having retained both polymerase activity and 3’-5’ exonuclease activity, it is irreversibly denatured from the high temperatures used to separate the new strands of DNA (4). The new enzyme must be manually added after every cycle – for the typical 30-40 cycles in PCR, this poses a contamination risk and is labour- and time- consuming. The Klenow fragment has since been replaced by thermostable enzymes from thermophilic bacteria species¬, which do not require addition of new polymerase between cycles (4).
Check out our collection of mesophilic DNA polymerases here.
PCR buffer components and thermal cycling conditions can affect DNA polymerase fidelity in different ways or to different degrees. For example, while magnesium ions are required as co-factors and they help guide DNA polymerase selection for the correct nucleotide, excess magnesium will reduce the fidelity of DNA polymerases. Unequal nucleotide concentrations will also decrease fidelity as mis-incorporation of the higher concentration nucleotide will occur more frequently. Thermal cycling conditions such as heating the DNA template to high temperatures for extended duration can lead to the release of bases from the phosphodiester backbone, leading to lowered fidelity.
Processivity
In addition to fidelity, DNA polymerases can be evaluated by their processivity, which is the number of nucleotides a polymerase is able to incorporate before dissociating (9). A polymerase with high processivity binds to the template and extends far and possibly to the end, adding several nucleotides per second. However, most DNA polymerases are intrinsically low in processivity, that they frequently bind to the template and dissociates, adding one nucleotide per second and producing short DNA product per association/dissociation event (9). In nature, a processivity factor called DNA clamp is commonly found in organisms to assist the DNA polymerase, to facility timely replication of the large genome. DNA clamps are multimeric proteins in the shape of a ring. It slides along the DNA strand, and its protein-protein interaction with DNA polymerase helps the association between the polymerase and the DNA strand. DNA polymerases with DNA clamps have dramatically higher processivity, capable of polymerizing thousands of nucleotides without dissociating from the DNA template. The scientist also developed DNA polymerases with high processivity by fusing a DNA binding domain to the polymerase, thus maximizing PCR yield and speed, especially for long templates amplification. (31) (32) Processivity is also affected by the buffer conditions such as salt concentration and the sequence of the DNA template(33).
Extension rate refers to the speed at which the nucleotides are added per molecule of DNA polymerase during extension, and is proportional to the processivity of the DNA polymerase. Extension temperature, buffer conditions, and template sequences can affect the extension rate, and a higher extension rate generally leads to reduced thermal cycling time.
Finally, thermal stability is also essential for DNA polymerases to maintain its stabilities at high temperature in PCR. It can be measured by the half-life of DNA polymerases to retain its activity under sustained temperature as high as 95°C. It is intimately related to the fidelity and processivity attributes of the DNA polymerase. (34)
Abm'sTaqFast DNA polymerase has an outstanding extension speed of 4-6kb of DNA per minute, making it suitable for quick PCR experiments.